3. Results and Discussion
3.1. Morphological Characteristics of the Bacteriophage Virions Via TEM Analyses
TEM photomicrographs of phages SentS01L and SentS01T are displayed in Figure 1.
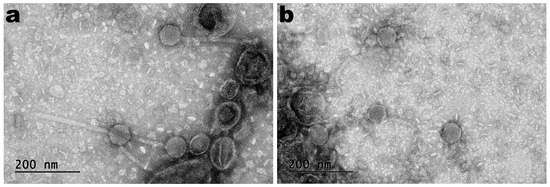
Figure 1. Negative staining TEM photomicrographs of phages SentS01L (a) and SentS01T (b).
The phages’ morphology (Figure 1) indicates that both phages exhibited siphovirus morphotypes and were classified under the Caudoviricetes class. Phages SentS01L and SentS01T displayed flawless icosahedral heads and lengthy, flexible tails that were not contractile.
3.2. Structural Protein Profile of Phage Sents01l and Sents01t Virions Obtained through SDS-PAGE Analysis
The results of SDS-PAGE electrophoresis (Figure 2) show that both phages had a structural protein profile across a broad range of molecular weights.
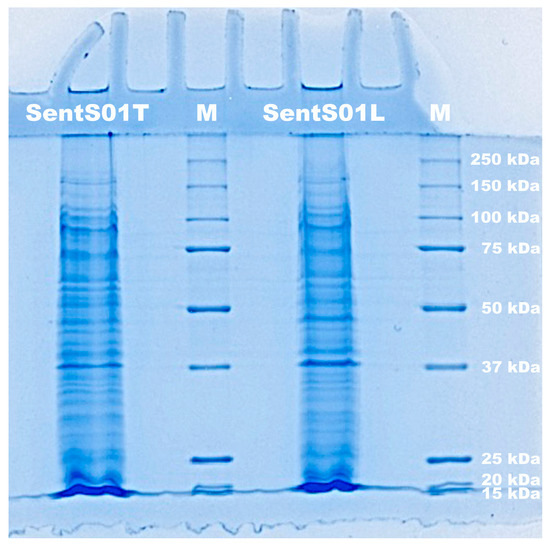
Figure 2. Electrophoretogram of the proteins of phages SentS01L and SentS01T and of molecular weight markers (lane M).
3.3. Preparation and Characterization of the Edible Antibacterial Coating (Ediphage) Integrating the Lytic Bacteriophage Cocktail
The edible antibacterial coating was prepared with sodium alginate via ionotropic gelling. Alginate is a polysaccharide of natural origin, which shows interesting characteristics, including its non-toxicity, biodegradability, and gelling ability [31]. Hence, it was chosen for the formulation of the edible antibacterial coating. Sodium alginate has been effectively used in edible coatings and films to protect food as well as to serve as a carrier for certain food preservation agents (antioxidants and antimicrobials) [32]. Moreover, additional research has suggested that matrices based on alginate are appropriate for phage inclusion [33]. Using CaCO3 in the alginate solution, as it is poorly soluble, allowed a controlled and uniform gel formation.
The technique allowed for the preparation of a coating with a film-like appearance, thickness, and flexibility suitable for use as an antibacterial coating for food applications. The coating was uniformly translucent and allowed the formation of a delicate film on the cheese samples (Figure 3a,c) with a thickness of 0.03 mm ± 0.009 (EdiPhage coating) and 0.03 mm ± 0.005 (phage-free coating). After a period of 20 h, the film remained unchanged, indicating that the addition of the phage cocktail did not alter the three-dimensional structure of the gel (Figure 3d).
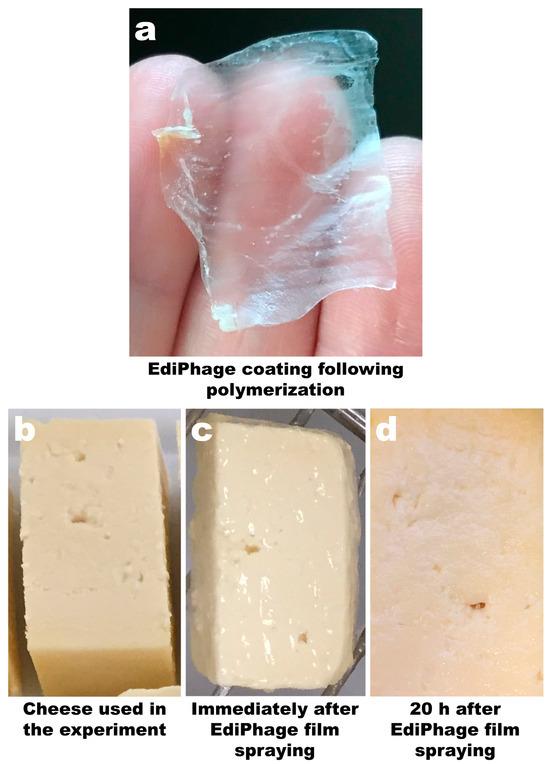
Figure 3. Image of the calcium alginate EdiPhage obtained via ionotropic gelling (a) and images of ripened cheese samples before spraying with the EdiPhage formulation (b), immediately after spraying with the EdiPhage formulation (c), and 20 h after spraying with the EdiPhage formulation (d).
Since phage particles’ availability and/or vitality might be impacted by their immobilization on various matrices, the activity of the phage virions immobilized in the EdiPhage coating was assessed. The process of integrating them into films, coatings, and hydrogels exposes them to stress conditions including stirring, drying, and mixing [34].
Images of Petri plates with S. enterica CCCD-S004 and samples of the Ediphage coating with and without the cocktail of lytic phages are shown in Figure 4a,b. Lysis zones are visible in Figure 4b, suggesting that the phage cocktail’s lytic activity is maintained after the EdiPhage formulation polymerizes. The phage virion particles’ entrapment within the EdiPhage coating’s polymerized calcium alginate biopolymeric matrix made possible the virions’ structural and functional stability while preserving their lytic viability.
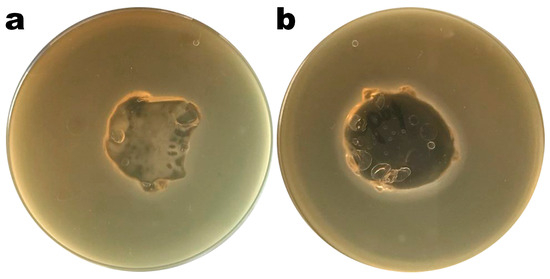
Figure 4. Results from assessment of the lytic viability of entrapped bacteriophage particles within the polymerized EdiPhage coating. (a) EdiPhage matrix devoid of phage particles and (b) bioactive EdiPhage coating integrating the phage cocktail at MOI 1000.
Regarding the assessment of the cytotoxicity of the bioactive antibacterial EdiPhage coating, the results indicate that there was no cell death whatsoever caused by contact with the EdiPhage coating after 24 h in the assay performed with either HaCaT or 3T3 cell lines (Figure 5).
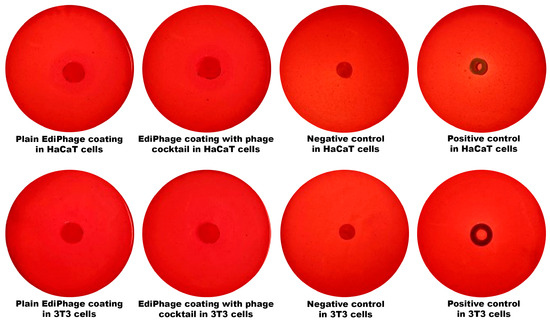
Figure 5. Analysis of the cytotoxicity with HaCaT and 3T3 cell lineages of the antibacterial EdiPhage coating integrating the cocktail of lytic phage particles.
The elemental compositions of the EdiPhage coatings devoid of bacteriophage cocktail and integrating the bacteriophage cocktail were determined using an X-ray fluorescence spectrometer. The most common substances were cellulose [(C12H20O10)n], sodium (Na), chlorine (Cl), and calcium (Ca) (Figure 6). Sodium alginate, the basis of the edible coating, is extracted from seaweed, and, in its extraction and purification process, formaldehyde, 0.2% HCl solution and 2% sodium carbonate solution are normally added [35]. Therefore, cellulose, sodium, and chlorine—in larger amounts—are derived, most likely from sodium alginate. Because calcium (Ca) was added during the ionotropic gelation process, it was also discovered in larger concentrations. Phosphorus (P), potassium (K), and magnesium (Mg) were additional notable elements, most likely originating from the phage suspensions. But these substances are not thought to be harmful. The other elements present, but in lower concentrations, probably originated from the raw materials used to obtain the EdiPhage coating. The elements may be present naturally, purposefully added during the synthesis of the product, or accidentally introduced as a result of interactions with processing machinery throughout the manufacturing process [36].
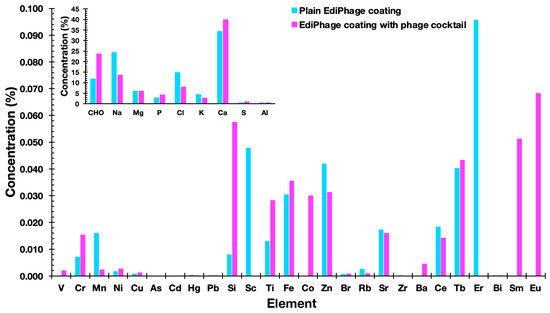
Figure 6. Elemental profiles of the plain EdiPhage coating and of the antibacterial bioactive EdiPhage coating integrating the phage cocktail, obtained via EDXRF.
Figure 7 shows the FTIR spectra of the plain EdiPhage coating, the antibacterial bioactive EdiPhage coating integrating the phage cocktail, and the phage cocktail in SM buffer.
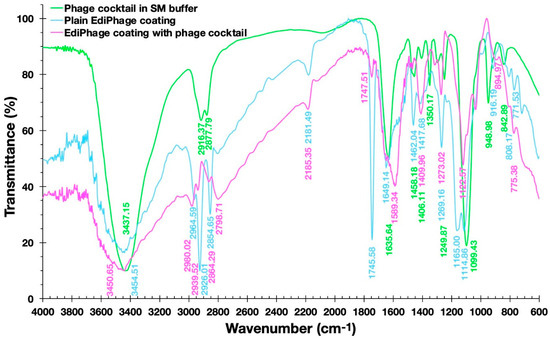
Figure 7. Fourier-transform infrared (FTIR) spectra of the plain EdiPhage coating (blue line), of the antibacterial bioactive EdiPhage coating integrating the phage cocktail (magenta line), and of the phage cocktail in SM buffer (green line).
Fernandes et al. [37], analyzing a sodium alginate-based polymer, observed a broad band between 3200 and 3600 cm−1 corresponding to the elongation of the OH groups present in the alginate polymer chain. The same was observed by Daemi and Barikan [38] between the bands between 3000 and 3600 cm−1 (Figure 7). In the spectra obtained, wide bands around 3500 cm−1 can also be observed. Fernandes et al. [37] also observed bands at 1414 cm−1 and 1621 cm−1, which were correlated, respectively, with the asymmetric and symmetric axial deformations of the -COO- groups, indicating the presence of a carboxylic acid group in the alginate. In the studied spectra, more intense peaks were observed in the plain EdiPhage coating (without phage particles) in the approximate range between 1700 and 1100 cm−1, particularly in the peaks at 1745.58 and 1165.00 cm−1 (Figure 7). Perhaps there could be some kind of interaction between the compounds arising from the SM buffer and the -COO- groups. Helmiyati and Aprilliza [39] observed a peak around 2928 cm−1 in an alginate sample and indicated that it was due to the stretching of the CH2 group. A close peak (2926.01 cm−1) was observed in the plain EdiPhage coating (without phage particles) (Figure 7). The absence of this peak was found in the antibacterial bioactive EdiPhage coating integrating the phage cocktail (Figure 7), which might indicate some kind of interaction between the compounds arising from the SM buffer and the alginate, such as CH-O bonding. Because of its very poor electronegativity when compared to oxygen and nitrogen, carbon is not considered a conventional traditional hydrogen bond donor. Nevertheless, several investigations have demonstrated that even aliphatic carbon atoms are capable of forming weak hydrogen bonds, which are designated as CH-O hydrogen bonds [40].
The bands occurred at 2964.59 and 2798.71 cm−1 (bioactive EdiPhage coating integrating the phage cocktail) and 2964.59; those at 2926.01 and 2854.65 cm−1 (plain EdiPhage coating (without phage particles)) can be attributed to aliphatic C-H vibrations [38]. These bands in the antibacterial bioactive EdiPhage coating integrating the phage cocktail were less evident, perhaps also due to CH-O bond formation. The presence of phage cocktail was irrelevant, probably related to the low percentage used to produce the EdiPhage coating.
In the characterizations of both the plain and bioactive EdiPhage coatings, thermal analyzes were also carried out. The DSC thermograms of the two EdiPhage polymeric coatings are displayed in Figure 8.
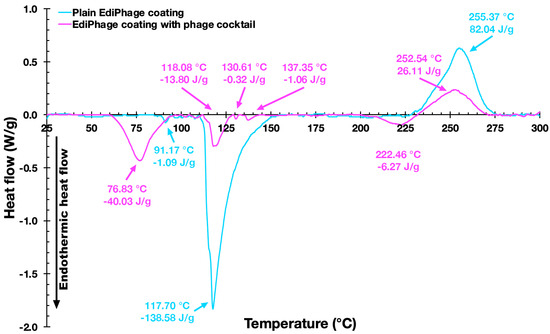
Figure 8. Differential scanning calorimetry thermograms of the EdiPhage coating devoid of phage particles (blue line) and of the EdiPhage coating integrating the cocktail of phage particles (magenta line).
Figure 8 shows thermograms of the EdiPhage coating. The first endothermic events (sample and control) are probably related to coating dehydration [41]. The first endothermic events (76.67 °C/−35.79 J/g (sample) and 91.17 °C/−1.05 J/g control)) are probably related to the evaporation of hydration water molecules from the films [41]. The endothermic peak related to the dehydration process occurs at around 80 °C in sodium alginate [42]. The second endothermic events (118.08 °C/−8.41 J/g (sample) and 117.70 °C/−150.11 J/g (control)) may perhaps be due to a depolymerization process with a formation of carbonaceous residue [42,43]. The exothermic events (252.37 °C/49.43 J/g (sample) and 255.37 °C/80.91 J/g (control)) probably corresponded to cleavage enthalpies such as the breakage of bonds within the complex [44]. The sample also showed two small endothermic events, which might be due to the influence of components in the buffer solution (where the phages are diluted) that increase the conformational stability through the electrostatic interactions of the present components [45]. According to Helmiyati and Aprilliza [39], thermal analysis tests with alginates demonstrated that the decomposition temperature of pure sodium alginate was 251.12 °C, which is very close to the degradation temperature of the prepared coatings (sample and control), as can be seen in the exothermic events. From the results presented, it can be said that the coatings are stable for the intended application.
The three-dimensional images obtained via X-ray transmission tomography (XRT) (Figure 9) allow the uniformity and homogeneity of the EdiPhage coating to be observed. There is no zone of greater or lesser atomic density (Figure 9), in general, which is interesting for a food-grade coating.
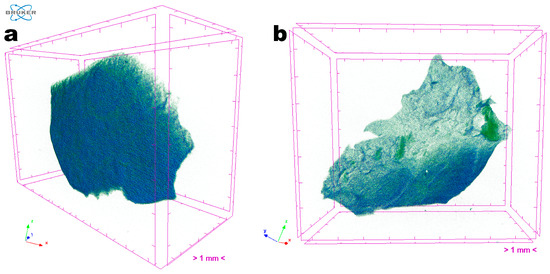
Figure 9. Images obtained through tomographic analyses via X-ray transmission of the EdiPhage coating loaded with phage particles: (a) front view of an EdiPhage sample and (b) slant view of the same EdiPhage sample. Three-dimensional image slices were gathered using an operating voltage set at 31 kV and electric current with 661 μA.
Images obtained via scanning electron microscopy (SEM) of the surface of the polymerized antibacterial EdiPhage coating confirmed its homogeneous characteristics (Figure 10).
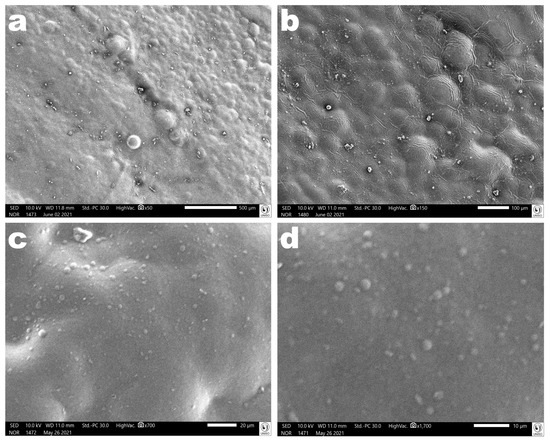
Figure 10. Photomicrographs of the polymerized antibacterial EdiPhage coating surface at several magnifications ((a): ×50, (b): ×150, (c): ×700, (d): ×1700). Images obtained via scanning electron microscopy (SEM) confirmed the formation of a coating with homogeneous characteristics.
The results obtained in the evaluation of the mechanical properties of the polymerized EdiPhage coating are displayed in Figure 11. In general, it can be said that the mechanical properties are directly linked to the degree of the crosslinking of the polymeric network, in addition to the characteristics of the alginate itself (molecular weight and M/G ratio (β-d-mannuronic acid (M blocks) and α-L-guluronic acid) (G blocks)) [46,47]. At the molecular level, the presence of Ca2+ ions is considered an obstacle to the rotation (movement) of alginate chains, decreasing their mobility and, consequently, the film’s ability to elongate [48]. Both the plain EdiPhage coating and the antibacterial EdiPhage coating integrating the cocktail of phage particles showed calcium contents close to each other. More elastic films have lower tensile strengths, with a consequent reduction in the maintenance of integrity [49]. Polysaccharide-based edible coatings and films are tough, highly soluble, colorless, and flexible because polysaccharides have linear structures. Edible coatings based on sodium alginate, normally, show good film-forming properties and are one of the most popular biodegradable polymers [50,51]. The mechanical properties are strongly influenced by the film formulation [52]. Accordingly, the range can be wide depending on the percentage of hydrocolloids added [53].
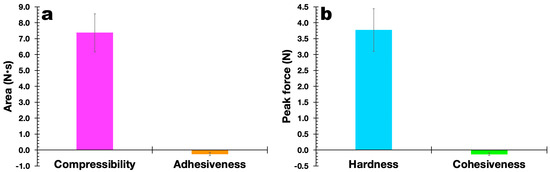
Figure 11. Results obtained for the compressibility and adhesiveness (a) and hardness and cohesiveness (b) of the antibacterial EdiPhage coating produced, integrating the cocktail of lytic bacteriophage particles.
The results obtained allowed us to confirm the suitability of the formulation for the intended purpose. The hardness of the EdiPhage coating formulated was ca. 3.75 N, which is suitable for the intended use. A lower hardness makes the EdiPhage coating less brittle, and therefore, the chances of premature detachment from the surface of the product are low. Since the antibacterial EdiPhage coating is meant to package ripened cheese while allowing the release and mobility of the phage particles, the low value obtained for the adhesiveness of the coating endowed with antibacterial properties will exert a significant influence on the availability of the lytic phage particles in the intended application site. Given that the coating was created by polymerizing alginate polysaccharide, the greater value for the compressibility of the antibacterial EdiPhage coating was consistent with the formulation’s less-than-half-solid state. The release of phage particles at the product’s surface is crucial, and the poor cohesiveness generated (Figure 11) is consistent with the low adhesiveness value. Therefore, the outcomes in terms of (a not-so-high) hardness, higher compressibility, poor adhesiveness, and low cohesiveness are all consistent with the intended use of the antibacterial EdiPhage coating. Given that cohesiveness and adhesiveness are crucial qualities for food applications, the antibacterial EdiPhage coating’s capacity to adhere to the surface of the matured cheese, but not to a higher extent, is an extremely significant property. The capacity of the biopolysaccharide to establish bonds in polymer chains, which results in resistance to their separation when subjected to mechanical pressures, is primarily responsible for the mechanical qualities of the EdiPhage coating [54,55]. Therefore, the mechanical resistance criteria evaluated were compressibility, hardness, adhesiveness, and cohesiveness; resistance to traction, relaxation, and resilience properties were deemed irrelevant for the produced antibacterial EdiPhage coating. Hence, based on the data given here, the antibacterial EdiPhage coating can preserve its physical integrity.